Research
Our research focuses on the physics and chemistry of quantum solid state systems, which emerge in van der Waals heterostructures built from atomically thin 2D crystals. It can be broadly separated in two areas: studies of electronic and optical properties of van der Waals heterostructures (for instance, twistronics), and observation of chemical reactions in liquids with atomic resolution electron microscopy.
An essential component shared by both research directions is the novel nanofabrication methods we develop for proof-of-principle prototyping and small-scale manufacturing aiming to advance quantum technologies, low power electronics, and catalysis.
Advanced Nanofabrication
We focus on developing novel nanofabrication techniques to produce high-quality van der Waals heterostructures, assembled layer-by-layer with ultra-sharp and ultra-clean interfaces.
​​
For this purpose, we have developed a pioneering ultrahigh vacuum (UHV) multi-chamber cluster tool, allowing the assembly and characterisation of van der Waals heterostructures in UHV environments.
​​​
To create functional devices from these van der Waals heterostructures, we utilise state-of-the-art equipment in our ISO class 5 and 6 cleanrooms at the National Graphene Institute.
Twistronics
Introducing twist between 2D crystals with similar lattice constants gives rise to moiré superlattices—a periodic variation of a local atomic registry, which in turn causes modulation of interlayer hybridisation, symmetry and strain, unlocking a new way to tune the electronic and optical properties of the heterostructures.
We recently demonstrated that atomic lattices of transition metal dichalcogenides (TMDs) undergo substantial reconstruction for small enough twist angles (θ<2°). As a result, instead of gradually changing atomic displacement between the lattices, perfectly stacked bilayer regions (or domains) form, which possess lower adhesion energy between the layers compared to that of two twisted lattices. Such lattice adjustment is accompanied by the strain which accumulates within narrow domain boundaries, resulting in a periodic domain network separated by a network of dislocations.

STEM image of triangular moiré lattice on MoS2/MoS2. Commensurate domains outlined in white.
Liquid Cells for In-situ Electron Microscopy
Another direction of our research is the application of 2D materials for atomic resolution imaging of chemical processes in liquid environment. Transmission electron microscopy (TEM) is a powerful tool for imaging and characterising materials at the nanoscale, but the high vacuum inside the microscope column usually limits the nature of the observable samples. While the majority of application-relevant chemical processes take place in liquids or gases, studying them in TEM presents a challenge.
Liquid cells employ two electron transparent but impermeable membranes to contain a liquid sample, preventing it from evaporating under the high vacuum environment of the electron microscope and enabling dynamic imaging of liquid samples. However, the thickness of the windows and the liquid layer generates increased electron scattering, limiting the ultimate resolution. By replacing commercially available silicon nitride windows (20 – 50 nm thick) with graphene windows, we can reduce the window thickness to below 1nm, dramatically reducing electron scattering and therefore improving imaging ability.
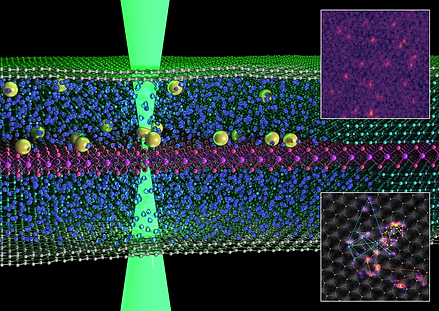
Two hBN spacer layers with a MoS2 monolayer (purple) sandwiched between them. Both hBN spacers contain voids pre-patterned using lithography and reactive-ion etching. Liquid samples are trapped inside the voids using few-layer graphene (FLG) on the top and bottom of the stack (green). The atomically flat hBN crystals form a hermetic seal with graphene and MoS2. Inset top right: HAADF-STEM image. The probe is focused on the submerged MoS2 layer so that the Mo lattice and Pt adatoms are clearly visible. Inset bottom right: A single Pt adatom trajectory from a 134 s video in the graphene double liquid cell.